UG105: Obvious: The Solution to the Dark Matter puzzle
David Noel
<davidn@aoi.com.au>
Ben Franklin Centre for Theoretical Research
PO Box 27, Subiaco, WA 6008, Australia.
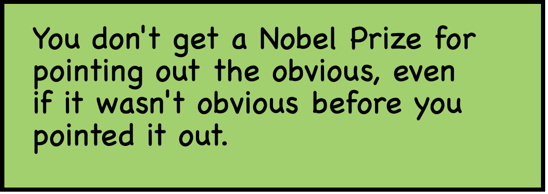
Quotation UG105-Q1.
What is Dark Matter?
Dark Matter is a substance postulated by the brilliant Swiss-American astrophysicist Fritz Zwicky around 90 years ago. It was
put forward to explain movement of galaxies which appeared not to obey known gravitational laws. Zwicky described the properties of Dark Matter, but left its nature and origin as a puzzle for later solution. Since then, many explanations have been put forward, some quite exotic or strange, but till now none has survived rigorous scrutiny.
The situation is described as follows in [4].
"Dark matter was postulated by Swiss astrophysicist Fritz Zwicky of the California Institute of Technology in 1933. He applied the virial theorem to the Coma cluster of galaxies and obtained evidence of unseen mass. Zwicky estimated the cluster's total mass based on the motions of galaxies near its edge and compared that estimate to one based on the number of galaxies and total brightness of the cluster. He found that there was far more estimated mass than was visually observable.
This is known as the "missing mass problem." Based on these conclusions, Zwicky inferred that there must be some non-visible form of matter, which would provide enough of the mass and gravity to hold the cluster together. Other observations have indicated the presence of dark matter in the universe, including the rotational speeds of galaxies, gravitational lensing of background objects by galaxy clusters such as the Bullet Cluster, and the temperature distribution of hot gas in galaxies and clusters of galaxies."
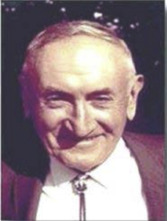
Fig. UG105-F1. Fritz Zwicky. From [4].
Wikipedia explains the situation as follows.
"Dark matter is a hypothetical form of matter thought to account for approximately 85% of the matter in the universe [3]. It's called "dark" because it does not appear to absorb, reflect, or emit electromagnetic radiation and is, therefore, difficult to detect.
Various astrophysical observations -- including gravitational effects which cannot be explained by currently accepted theories of gravity unless more matter is present than can be seen -- imply dark matter's presence. For this reason, most experts think that dark matter is abundant in the universe and has had a strong influence on its structure and evolution. The primary evidence for dark matter comes from calculations showing that many galaxies would behave quite differently if they did not contain a large amount of unseen matter.
Some galaxies would not have formed at all, and others would not move as they currently do. Other lines of evidence include observations in gravitational lensing and the cosmic microwave background, along with astronomical observations of the observable universe's current structure, the formation and evolution of galaxies, mass location during galactic collisions, and the motion of galaxies within galaxy clusters.
No one has directly observed dark matter yet, primarily because it doesn't usually interact with ordinary (baryonic) matter or radiation except through gravity. Dark matter may be composed of some as-yet-undiscovered subatomic particles. A leading candidate for dark matter has been a new kind of elementary particle that has not yet been discovered, such as weakly interacting massive particles (WIMPs), or axions. Other possibilities include black holes such as primordial black holes. Many experiments to detect and study dark matter particles directly are being actively undertaken, but none have yet succeeded.
Although the scientific community generally accepts dark matter's existence, some astrophysicists argue for various modifications of the standard laws of general relativity. These include modified Newtonian dynamics, tensor-vector-scalar gravity, or entropic gravity. However, so far none of the suggested models can correctly describe all observed phenomena at once."
So, until now the Dark Matter puzzle has seemed complicated and requiring advanced science to solve. But the current article shows that the answer to the puzzle is simple and obvious, based on well-known and accepted evidence.
Understanding types of galaxy
From around the 1940s, more and more understanding was gained of the nature and classification of the various types of galaxy existing beyond our own galaxy, the Milky Way.
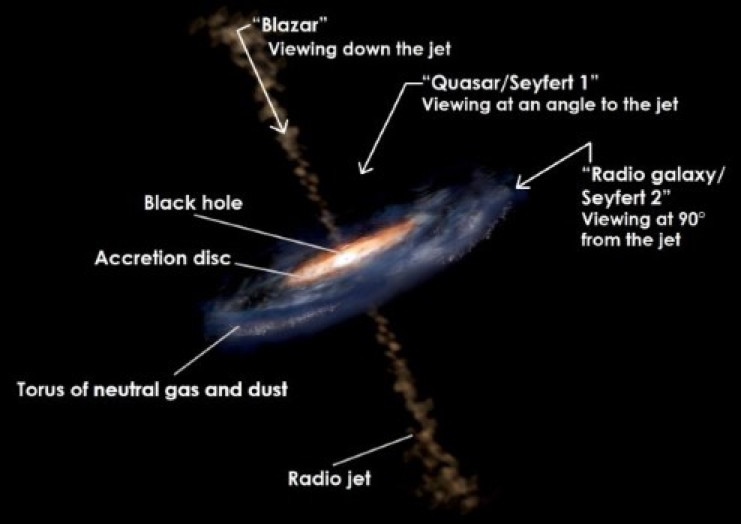
Fig. UG105-F2. Viewing of an AGN from different angles. From [2].
According to their appearance and properties, galaxies were assigned names such as Quasars, Seyfert Galaxies, Radio Galaxies, and Blazars. It was subsequently realized that all these names were being applied to the same objects, but viewed from different angles (see Figure F2).
At the heart of every galaxy is an "AGN", an Active Galactic Nucleus. This is a rapidly spinning object, also described as a Supermassive Black Hole, with a mass millions, or even billions, times that of the Sun. Some properties of AGNs are known, but their exact nature is still subject to considerable controversy.
All AGNs emit very powerful beams of electromagnetic radiation along their axes of rotation, and the more massive ones also emit beams of particles, such as protons (hydrogen nuclei), electrons, and helium nuclei. The mechanism of generation of these beams is still uncertain, but one of their main properties is that they are very tightly collimated -- contained in a constrained beam showing minimum spread -- rather like a laser beam.
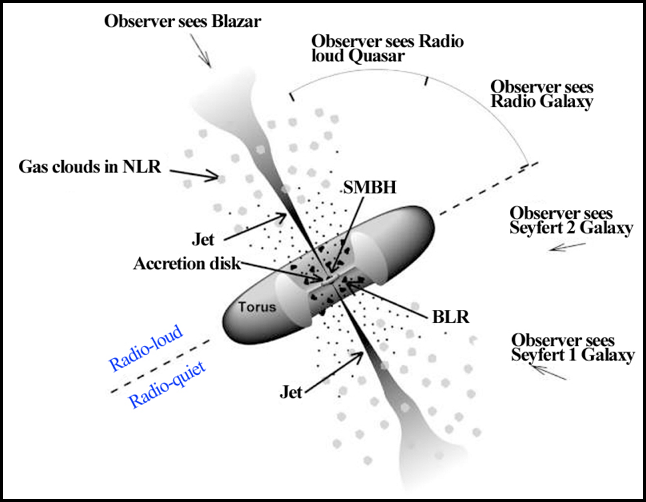
Fig. UG105-F3. Diagram of AGN jet observation. From [1].
Viewing AGN axial beams
Because the axial beams are are so narrowly constrained, they are only obviously visible when viewed head on, very close to the beam axis itself. Observers off the axis only see the much weaker side effects, such as scattering of the beams when they encounter interstellar gases or dust.
Figure F3 shows how an observer may differently interpret the same galactic object according to their position relative to the AGN's axial beam. Viewed head-on, within a few degrees of the axis, the galaxy is very bright, and is classed as a Blazar.
And here is the simple and obvious origin of the Dark Matter concept. When viewing an AGN, it is only seen in visible light when the observer is right on the beam axis. Away from this axis, the AGN appears dark.
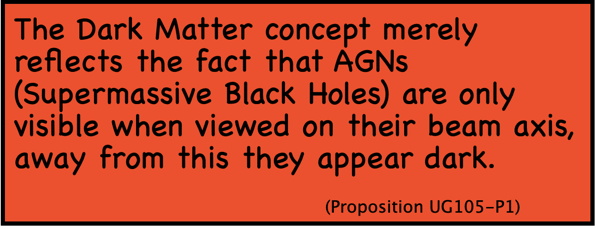
Proposition UG105-P1.
Relativistic Jets
The Figure F3 diagram also depicts so-called Relativistic Jets emanating from the AGN. These are not the axial beams themselves, only the side effects of these beams if they happen to encounter dust or other scattering action on their path. The jets are called "relativistic" because they contain particles moving at significant fractions of the speed of light, and as such their behaviour varies from the everyday according to the theory of relativity.
It will be apparent that how and whether these jets show up depends on just what the axial beams encounter on their path.
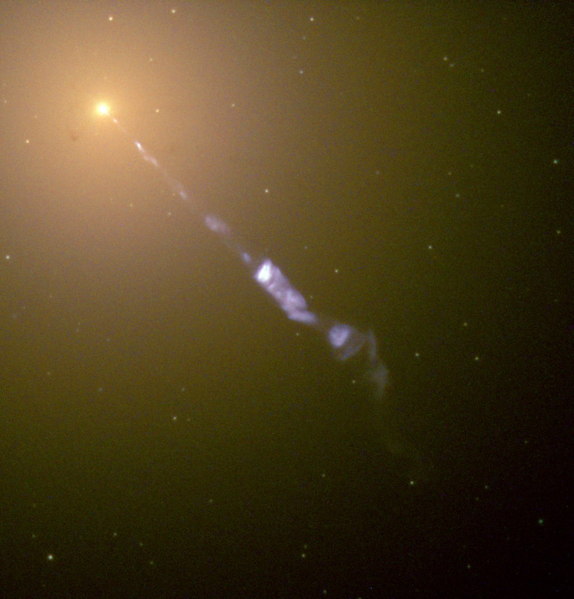
Fig. UG105-F4. Relativistic jets from the M87 Galaxy axial beam. From [7].
Figure F4 shows the AGN at the center of the Messier 87 Galaxy ejecting a jet of superheated matter from one of its poles [7]. It is a composite photograph, combining images taken at different wavelengths by the Hubble Telescope. The jet extends over about 5000 light-years (as a comparison, the nearest star to our own Sun is about 4 light-years away). The original caption for Figure F4 was "Black Hole-Powered Jet of Electrons and Sub-Atomic Particles Streams From Center of Galaxy M87" [7].
M87 is one of the biggest and closest elliptical galaxies [8]. It is located about 55 million light-years away from Earth, in the constellation Virgo. For comparison, the nearest companion galaxy to our Milky Way, the Andromeda Galaxy, is about 2.5 million light-years away.
The spread of Axial Beams
Although the axial beams from AGNs are tightly collimated, like laser beams, they will show some degree of spread. This is important in the Dark Matter context, because the spread will affect how much of an AGN's output is seen as dark, how much light. The spread of AGN axial beams is not well understood at present -- it may be different for different-mass AGNs.
Looking again at Figure F4, the jet does appear to show spreading, but this may due to perspective. However, it is noted in [7] that the M87 galaxy is too distant for the Hubble Telescope to resolve individual stars, and the bright dots in the image are star clusters, assumed to contain some hundreds of thousands of stars each. According to [9], globular star clusters contain anywhere from tens of thousands to millions of stars, packed tightly together in dense clumps ranging from 50 to 450 light-years across. So, over its length of 5000 light-years, the beam has apparently spread to a width of 50 or more light-years.
Why so long to understand Dark Matter?
Given the simple and logical nature of the present solution to the Dark Matter Puzzle given here, it might be asked, "Why has it taken 90 years to be found?".
There were a number of factors involved here, but two of the most important were the Schwarzschild Radius / Event Horizon misapplication and the lack of understanding of Vortex Stars.
In 1915, Karl Schwarzschild made some calculations about a theoretical entity called a "Black Hole", using field equations published that same year by Albert Einstein. He showed that when a Concept Black Hole of the type he studied had a large enough mass, its gravitational force was so great, that nothing could escape from it, not even light.
From this grew a persistent and widespread belief that Black Holes could not emit any form of radiation or matter. Both AGNs and Stellar Black Holes (one of the results of ordinary star end-of-life blowup) are classed as Black Holes. The evidence above mostly concerns routine emissions of radiation and particles from Black Holes. How can this be?
The thing is, Schwarzschild's Concept Black Holes are defined as non-rotating bodies, whereas Real Black Holes rotate, and do so very rapidly, The Schwarzschild calculations apply to a theoretical body not known to exist in the real world.
The second major restraint on understanding the situation was the limited knowledge of the three classes of stars. The vast majority of stars visible with the naked eye from earth are in our own Milky Way galaxy. These stars generate and emit radiation powered by fusion of atomic nuclei. They can be called Fusion Stars.
All Fusion Stars have a specific lifetime, of a length depending on their mass (heavy stars die quickly, light ones live long). At the end of their life, Fusion Stars undergo Blowup. The ultimate result of Blowup depends on the mass of the star.
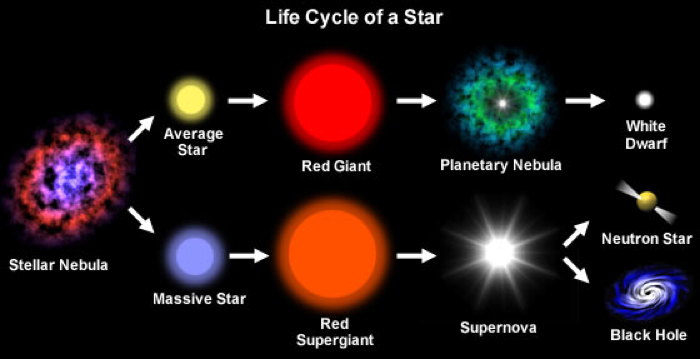
Fig. UG105-F5. Life Cycle of a Star. From [10].
Lighter stars end up as White Dwarfs, intermediate ones of around 3-8 times the mass of our Sun end up as Neutron Stars, and more massive stars end up as Stellar Black Holes. All these types are similar in nature, rapidly-rotating bodies like vortexes, and may be called Vortex Stars. AGNs form by the merger of smaller black holes and all sorts of other bodies, and are also Vortex Stars.
It has recently become apparent that with Vortex Stars, the greater their mass, the more rapidly they rotate,
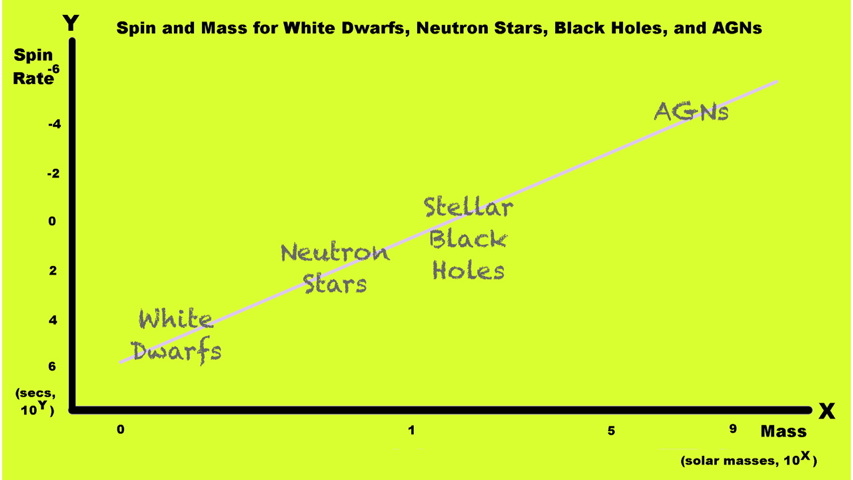
Fig. UG105-F6. Vortex Star rotation rates for various masses.
At the top end of the scale of Vortex Stars are Millisecond Quasars, bodies with a mass a billion times that of the Sun, and with rotation rates of hundreds of times a second. These lie well beyond ordinary objects of conception, and representations like those on Figures F2 and F3 are only diagrammatic attempts to give a feel for such objects.
Transition Stars are the various bodies which may be formed during Blowup, as a Fusion Star transitions into a Vortex Star. A division starts to appear between the outer half of the star, the Shell, and the inner half, the Core. The Core continues to contract, as its elements fuse, making a denser and smaller inner body. Because of conservation of rotational energy, the Core starts to spin faster and faster and continues its transformation to a Vortex Star.
The outer Shell expands and expands, first forming a Red Giant or Supergiant. At this point the Shell has no energy source within its own material, but contains the incipient Vortex Star, which emits an increasingly defined energy beam along its spin axis. While the Shell is whole, this energy beam strikes and is reflected around the whole Shell, finally escaping from the Shell surface as black-body radiation. As the total of black-body radiation depends directly on the area and temperature of the emitting surface, and because Transition Stars become huge in diameter, a Transition Star like Betelgeuse shows as a very bright object.
Eventually the Shell expands enough so that it breaks up, appearing as a planetary nebula or supernova, as in Figure F5. The process is slow for low-mass stars, for bigger-mass stars it may be very rapid.
Whether a visible vortex star is left after loss of the Shell to expansion depends on where the Core's spin axis happens to end up pointing. If the axis points toward the observer, the new star will be visible, if not, it will not be seen.
There is more detail on the processes involved in UG102: Understanding Vortex Stars: White Dwarfs, Neutron Stars, Black Holes, and AGNs [11].
Accretion Discs
Figures F2 and F3 each show a disc formed round the black hole, called an "accretion disc", said to represent matter
being drawn into the black hole ("accreted") from outside.
Accretion discs are fictional entities, invented to overcome the Schwarzschild limitation on radiation being emitted by a black hole -- instead of radiation coming from the black hole, it is supposed to come from the accretion disc.
There are no known observations evidencing the existence of accretion discs, nor is there physics to show why such a disc should emit radiation along its spin axis. But there is physics to show that any such disc could only be tiny, perhaps 100 km across -- a millisecond quasar would be rotating at 314,200 km/sec at its rim, faster than the speed of light. Not allowed in the real world.
The Fireworks Galaxy
The Fireworks Galaxy is a bright spiral galaxy located on the border between the constellations Cepheus and Cygnus. It is one of the nearest spiral galaxies to the Sun. It appears face-on, near the orange star Eta Cephei [5]. The galaxy has an apparent magnitude of 9.6 and lies approximately 25.2 million light years away. It has the designation NGC 6946 in the New General Catalogue.
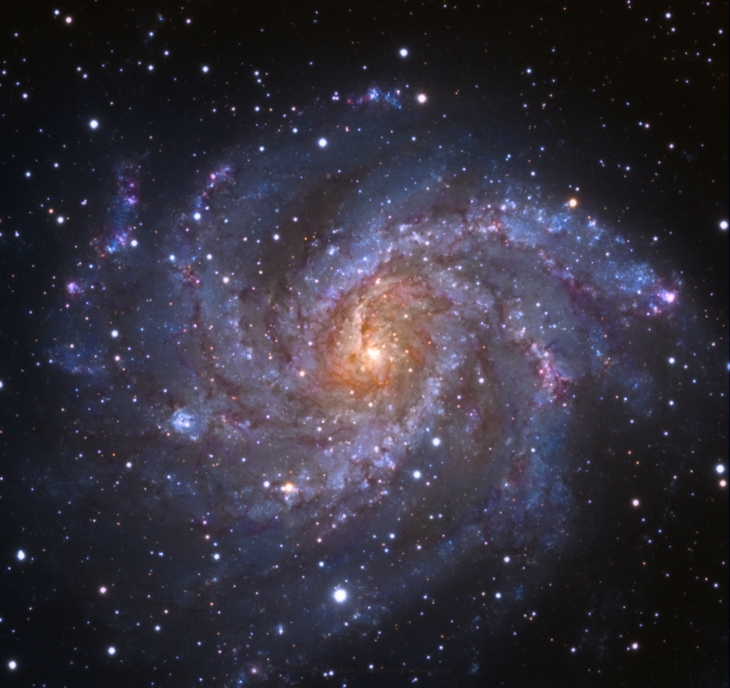
Fig. UG105-F7. The Fireworks Galaxy. From [6].
NGC 6946 was nicknamed the Fireworks Galaxy because it has hosted 10 supernovas over the last century, which is more than any other known galaxy and ten times the rate seen in the Milky Way [5]. It is classified as an active starburst galaxy, one that has an extraordinarily high rate of star formation. The Fireworks Galaxy is a popular target for astronomers studying the evolution of massive stars, as well as for amateur astronomers looking for bright targets for small and medium telescopes.
The Disappearing Supergiant
As well as for the high number of supernovas, the Fireworks Galaxy is also notable for hosting a disappearing star. This event was described in [5] as follows.
"The Fireworks Galaxy hosted a disappearing supergiant star, designated N6946-BH1, that is believed to have faded as a result of a failed supernova. The star's bolometric luminosity spiked to a million or more solar luminosities in the spring of 2009, but by 2015 the supergiant became invisible in optical wavelengths. The increase in luminosity was insufficient for a supernova and the star is believed to have collapsed to form a black hole. N6946-BH1 has been extensively studied because it provides evidence for the theory that exceptionally massive stars can collapse into black holes without undergoing supernova events.
N6946-BH1 was first detected in 2015. The team observed 27 galaxies using the Large Binocular Telescope for four years, in search of massive stars that had collapsed to form black holes without producing supernova events. The candidate, designated N6946-BH1, was reported to have an estimated mass between 18 and 25 solar masses.
In 2017, the team confirmed the optical disappearance of the star based on observations with NASA's Hubble Space Telescope. The star, a red supergiant with a mass about 25 times that of the Sun, had exhibited an outburst in 2009 during which it became more than a million times as luminous as the Sun. The Hubble images showed the star to be at least 5 magnitudes fainter in optical wavelengths, and its bolometric luminosity at least six times fainter than the progenitor's. Faint emission in the near-infrared band was detected that was believed to be associated with the source. The study suggested that the rapid decline was the result of a failed supernova caused by the core-collapse of a red supergiant star to a black hole.
The pre-collapse light curve showed a decrease in optical brightness starting in mid-2008, during which the star became noticeably redder. A 2019 study proposed that the progenitor was a yellow hypergiant with a surface temperature of about 5,000-6,000 K, that was experiencing high mass loss prior to the collapse. If the researchers are correct in concluding that the event was a failed supernova, this was the first time that black hole formation was observed".
Both these peculiarities of the Fireworks Galaxy, that is the fading of a (transition) star after blowup, and the apparent high number of supernovas being formed, are readily explained by what has been said above. The disappearance of a star after blowup is because the (vortex) star formed had its spin axis turned away from the observer.
The apparent high number of supernovas needs a little more explanation. A very obvious feature of the Fireworks Galaxy is that it is very close to face-on to the Earth, the axis of its galactic disc points straight towards us.
Galaxies, solar systems, and the like are subject to an influence called Equatorial Forcing (more detail at XT806: What is Equatorial Forcing? [12] ). Briefly, when a number of smaller bodies are associated with a central massive rotating body, the larger body acts gravitationally so the smaller ones move towards imitating the central body's equatorial plane and direction of rotation. This action, forming an aspect of Spin Gravity, in Einsteinian general relativity called "frame dragging", is the reason while solar systems and galaxies present as discs -- in the galaxy case, the central body is its AGN.
Equatorial Forcing not only influences solar systems in a galaxy to form into a disc, it also pushes the planes of individual solar systems towards the plane of the galaxy itself. In the case of the Fireworks Galaxy, the axes of rotation of all its stars, both fusion and smaller vortex stars, will have a preferential orientation towards us. Hence we see a much greater number of supernovas than from galaxies not face-on to us.
* * * * * * * * * * * * * * * * * * * *
References and Links
[1]. Number of obscured AGN compared to unobscured AGN. https://astronomy.stackexchange.com/questions/32493/number-of-obscured-agn-compared-to-unobscured-agn .
[2]. Chapter 14. Black Holes. http://www.thestargarden.co.uk/Black-holes.html .
[3]. Dark matter. https://en.wikipedia.org/wiki/Dark_matter .
[4]. Boris V. Alexeev, The Dark Matter Problem. https://www.sciencedirect.com/topics/physics-and-astronomy/coma-cluster .
[5]. Fireworks Galaxy (NGC 6946). https://www.constellation-guide.com/fireworks-galaxy-ngc-6946/ .
[6]. Fireworks Galaxy NGC 6946 - RGB Liverpool Telescope. https://www.astrobin.com/386537/C/ .
[7]. M87 Jet. https://commons.wikimedia.org/wiki/File:M87_jet.jpg .
[8]. First Ever 3D Map Of Messier 87 Galaxy Assembled. https://www.keckobservatory.org/m87/.
[9]. Chelsea Gohd. Star Clusters: Inside the Universe's Stellar Collections. https://universe.nasa.gov/news/235/star-clusters-inside-the-universes-stellar-collections/ .
[10]. Life Cycle of a Star. https://www.schoolsobservatory.org/learn/astro/stars/cycle .
[11]. David Noel. UG102: Understanding Vortex Stars: White Dwarfs, Neutron Stars, Black Holes, and AGNs. http://www.aoi.com.au/UG/UG102/index.htm .
[12]. David Noel. XT806: What is Equatorial Forcing?. http://www.aoi.com.au/Extracts/XT806.htm .
Go to the UG Home Page
Compilation started 2023 Sep 7. First version on Web, 2022 Sep 14.